DIII-D National Fusion Facility Completes Highest-Powered Negative Triangularity Experiments in History of US Fusion Research Program
- Configuration offers potential solutions for key fusion pilot plant design challenges.
San Diego, April 24. The DIII-D National Fusion Facility has completed an innovative month-long fusion research campaign utilizing a plasma configuration known as “negative triangularity.” The campaign was organized and conducted to explore the potential benefits of using this configuration to improve exhaust handling and particle confinement as a means of making future fusion energy plants more efficient and cost effective.
The campaign was a global collaboration and included the participation of researchers from 15 institutions. It produced the highest-powered negative triangularity experiments in the history of the US fusion research program.
Initial data from this work appear very encouraging and full results will be released in the summer of 2023 after further analysis.
The DIII-D National Fusion Facility
DIII-D is an Office of Science scientific user facility, operated by General Atomics (GA) for the US Department of Energy. Its mission is to pioneer the science and innovative technology that will enable the development of fusion as an energy source. DIII-D is the largest operating magnetic fusion research facility in the United States and one of the most flexible tokamaks in the world, which allows it to run innovative experiments and research campaigns that cannot be conducted anywhere else.
Fusion is the process that powers the stars and offers the potential for nearly limitless clean, safe energy. It occurs when two light nuclei combine to form a new one, releasing vast amounts of energy that can be used to generate electricity. Researchers can achieve fusion using a tokamak, a device that uses heat, magnetic fields, and microwaves to create and contain a plasma of charged particles. When enough heat is added, the plasma reaches fusion conditions.
Positive vs. Negative Configurations
To achieve fusion conditions relevant for energy production, DIII-D’s plasma must be heated to temperatures exceeding 100 million degrees Celsius—approximately ten times the temperature at the center of the sun. DIII-D generates these conditions inside a vacuum vessel that has a D-shaped cross-section. When operating with a standard configuration, the plasma inside the DIII-D tokamak takes the same “D” shape as the vacuum vessel. This is known as “positive triangularity” and is the configuration used in nearly every experiment at the DIII-D National Fusion Facility.
By comparison, the cross-section of a plasma configured in a “negative triangularity” appears mirrored, closely resembling a backwards “D” (Figure 1). Due to their shape within the fusion machine, plasmas in negative triangularity are less likely to impact the inner walls of the tokamak, potentially offering significant benefits for the design of future fusion power plants.
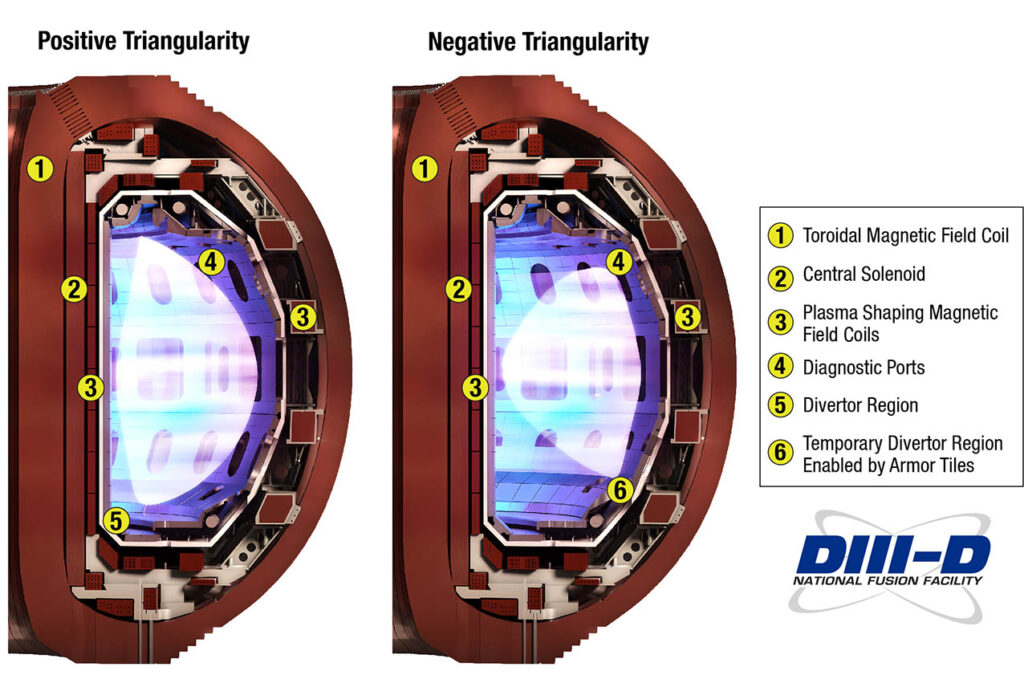
To prevent the harsh conditions within the tokamak from damaging the inner walls, DIII-D must channel excess heat away from the edge of the plasma. Using the machine’s magnetic fields, this heat exhaust is guided into a region known as the divertor, where it is efficiently dispersed, cooled, and vented. The divertor is a permanently installed component on DIII-D and is positioned for a standard plasma configuration.
Because negative triangularity plasmas place heat exhaust outside of the installed divertor region, DIII-D’s interior required a special modification. To enable the research campaign at DIII-D, the research team completed an engineering project to design and install special armor tiles to provide sufficient protection and create a temporary divertor region (Figure 2).
Designed to be one of the most flexible research tokamaks in the world, DIII-D is uniquely capable of accommodating changes to its interior and plasma configurations. Once the armor was in place, the campaign was able to run for four weeks in January and February 2023. The full month of experiments produced 178 run hours (22 percent of the 800 planned run hours throughout the calendar year).
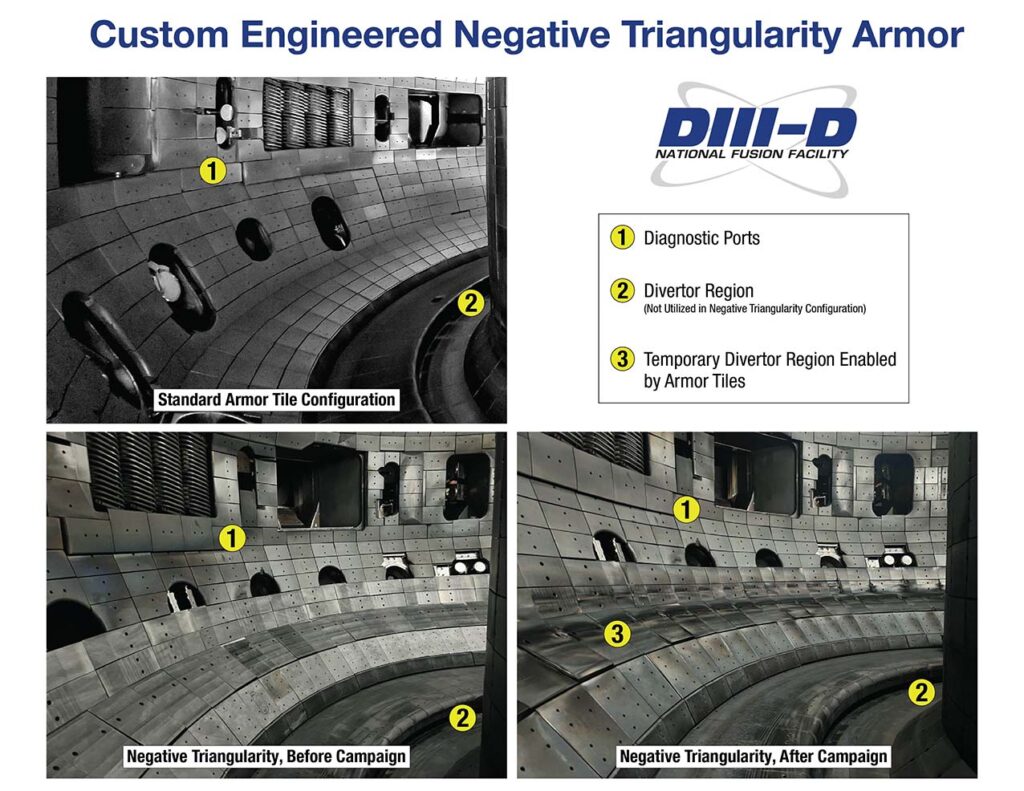
International Collaboration
Participants joined the research campaign from eight universities, four national laboratories, GA, and several international institutions, including the Swiss Federal Institute of Technology Lausanne, Germany’s Max Planck Institute for Plasma Physics, and Japan’s National Institute for Fusion Science.
“The negative triangularity research team deserves a lot of credit for their planning and execution of this research campaign,” said Dr. Richard Buttery, Director of the DIII-D National Fusion Facility. “As an Office of Science user facility, our goal is to deliver cutting-edge science for the world. This collaboration is a perfect illustration of what we can do when we bring different voices together from around the world to tackle a specific challenge.”
The research team was led by Dr. Kathreen Thome, a GA Scientist stationed at DIII-D, and Prof. Carlos Paz-Soldan, Associate Professor of Applied Physics at Columbia University’s School of Engineering. Prof. Paz-Soldan recently served as the co-lead for a collaboration between Columbia University and the Massachusetts Institute of Technology (MIT), facilitating a graduate-level fusion reactor design course in which students designed fusion reactors leveraging negative triangularity for electricity production.
“In my entire time working at DIII-D, I have never seen the research team come together like this,” said Dr. Thome. “From our incredible engineers who made this campaign possible, to our world-class operations and science groups, this was an international team effort from top to bottom. It was truly special to be part of it. I know the entire team is very excited to dive into the results.”
“Our campaign consisted of several experiments each targeting an important part of tokamak plasma physics, and our team benefited from great expertise on all fronts,” said Prof. Paz-Soldan. “We were able to gather data to push the limits of the plasma’s behavior in this unique configuration, and to understand how all the pieces fit together into a successful fusion reactor operating regime. It was an honor to help realize the campaign.”
Dr. Max Austin, a DIII-D Scientist affiliated with the University of Texas-Austin, served as the deputy research lead.
“This campaign was unique. Everyone involved contributed their own individual expertise, and it was great to see the team work together to solve challenges in real time and ensure successful experiments,” said Dr. Austin.